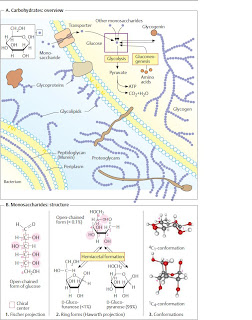
Overview of carbohydrates
The carbohydrates are a group of naturally
occurring carbonyl compounds (aldehydes
or ketones) that also contain several hydroxyl
groups. The carbohydrates include single sugars
(monosaccharides) and their polymers,
the oligosaccharides and polysaccharides.
A. Carbohydrates: overview
Polymeric carbohydrates–above all starch, as
well as some disaccharides–are important
(but not essential) components of food .
In the gut, they are broken down into
monosaccharides and resorbed in this form
. The form in which carbohydrates
are distributed by the blood of vertebrates is
glucose (“blood sugar”). This is taken up by the
cells and either broken down to obtain energy
(glycolysis) or converted into other metabolites
. Several organs (particularly
the liver and muscles) store glycogen as
a polymeric reserve carbohydrate .
The glycogenmolecules are covalently
bound to a protein, glycogenin. Polysaccharides
are used by many organisms as building
materials. For example, the cell walls of bacteria
contain murein as a stabilizing component
, while in plants cellulose and
other polysaccharides fulfill this role .
Oligomeric or polymeric carbohydrates
are often covalently bound to lipids or proteins.
The glycolipids and glycoproteins
formed in this way are found, for example,
in cell membranes (center). Glycoproteins
also occur in the blood in solute form(plasma
proteins; and, as components of
proteoglycans, form important constituents of
the intercellular substance .
B. Monosaccharides: structure
The most important natural monosaccharide,
D-glucose, is an aliphatic aldehyde with six C
atoms, five of which carry a hydroxyl group
(1). Since C atoms 2 to 5 represent chiral
centers , there are 15 further
isomeric aldohexoses in addition to D-glucose,
although only a few of these are important in
nature. Most natural monosaccharides
have the same configuration at C-5 as
D-glyceraldehyde–they belong to the D series.
The open-chained form of glucose shown
in (1) is found in neutral solution in less than
0.1% of themolecules. The reason for this is an
intramolecular reaction in which one of the
OH groups of the sugar is added to the aldehyde
group of the same molecule (2). This
gives rise to a cyclic hemiacetal . In
aldohexoses, the hydroxy group at C-5 reacts
preferentially, and a six-membered pyran
ring is formed. Sugars that contain this ring
are called pyranoses. By contrast, if the OH
group at C-4 reacts, a five-part furan ring is
formed. In solution, pyranose forms and
furanose forms are present in equilibrium
with each other and with the open-chained
form, while in glucose polymers only the
pyranose form occurs.
The Haworth projection (2) is usually used
to depict sugars in the cyclic form, with the
ring being shown in perspective as viewed
from above. Depending on the configuration,
the substituents of the chiral C atoms are then
found above or below the ring. OH groups
that lie on the right in the Fischer projection
(1) appear under the ring level in the Haworth
projection, while those on the left appear
above it.
As a result of hemiacetal formation, an additional
chiral center arises at C-1, which can
be present in both possible configurations
(anomers) . To emphasize this, the
corresponding bonds are shown here using
wavy lines.
The Haworth formula does not take account
of the fact that the pyran ring is not
plain, but usually has a chair conformation. In
B3, two frequent conformations of D-glucopyranose
are shown as ball-and-stick models. In
the 1C4 conformation (bottom), most of the
OH groups appear vertical to the ring level, as
in the Haworth projection (axial or a position).
In the slightly more stable 4C1 conformation
(top), the OH groups take the equatorial
or e position. At room temperature, each
formcan change into the other, as well as into
other conformations.